- 01 Overview
- 02 EMMA Science Highlights
- 03 How It Works
01 Overview
With EMMA (the Electromagnetic Mass Analyzer), TRIUMF scientists are creating exotic nuclei, many never before studied precisely in a laboratory, to provide insights into nuclear structure at the extreme and the origin of the elements formed in powerful stellar explosions.
EMMA is a recoil mass spectrometer, a tool designed to separate the heavy products of nuclear reactions (the recoils) from the beam that induces them. Like DRAGON, EMMA can measure the rates of nuclear reactions that occur in stars, but its design also permits the measurement of other reactions important for the study of nuclear structure.
A core part of TRIUMF’s astrophysics program, EMMA excels at sifting, sorting and detecting the recoils from a trio of nuclear reactions that take place in exploding stars, making the facility an ideal star simulator.
In nuclear transfer reactions, a small number of protons and/or neutrons are transferred between the projectile and target nuclei. In fusion-evaporation reactions, a projectile and target nucleus fuse into a single, excited nucleus that subsequently loses, or evaporates, a small number of protons, neutrons, and/or alpha particles. EMMA can also study radiative capture reactions, in which a nucleus fuses with a proton or alpha particle, and the excited recoil emits a gamma ray to reach a more stable energy level.
EMMA’s ability to mimic stellar nuclear reactions is underpinned by the fact that the facility can handle heavy ion beams of atomic mass of least 150 units, moving at up to a fifth the speed of light.
This makes EMMA a perfect testing ground for studying the nuclear reactions in exotic, high-energy cosmic events, such as Type 1 X-ray bursts. These occur when neutron stars gravitationally accrete hydrogen from a stellar neighbour and this hydrogen eventually detonates in fusion reactions on the neutron star’s surface.
Similarly, EMMA’s high-energy fusion-evaporation and transfer reactions are opening the door to research with new, exotic nuclei that occur in stars, but aren’t found naturally on Earth, such as 21Na. While providing insights into stellar processes, these exotic, short-lived nuclei are windows into the nature of nuclei near the limits of stability, and the character of the forces that holds the nucleus together.
02 EMMA Science Highlights
03 How It Works
Based in ISAC-II, EMMA scientists induce nuclear reactions by firing a heavy-ion beam at a thin-foil target. This results in a scattershot explosion of recoils of different energies, angles, momenta, masses and electric charges. EMMA is uniquely able to sift and sort this mélange of ions into a beam composed of, and focused solely based on, the recoils’ mass-to-charge ratio, all in less than a millionth-of-a-second.
EMMA, which from above looks like a pair of round rimmed glasses sitting on a nose arch, consists of five main parts along its 9.1 m length: the target; a series of quadrupole magnets; two electrostatic deflectors; a dipole magnet; and the focal plane detectors.
The heavy ion beam and target
An EMMA experiment begins with selecting an ISAC-II beam of radioactive heavy ions, for example rubidium-83 (83Rb). These are fired at EMMA’s target, a millionth-of-a-metre-thick foil, such as polyethylene, a good source of hydrogen, the most common element in the universe.
Moving at 10-20% the speed of light (speeds typical of nuclei in stellar explosions), the beam particles smash into the target, creating a mix of nuclear reactions and a scattershot explosion of ionized recoils, or reaction products, that exit the other side of the target film.
What’s notable is that the vast majority of the beam ions, more than 99.9%, pass right through the target foil without triggering fusion reactions. Thus, one of EMMA’s key tasks is separating the recoils from this river of unreacted beam ions.
Quadrupole doublets
The unreacted beam ions and recoils explode from the target in a cone-like spray, and must be focused back into a beam. This is accomplished by a pair of back-to-back quadrupole magnets that produce strong magnetic fields. The first quadrupole magnet is oriented to align the ions horizontally, the second aligns the ions vertically, creating a tight, pencil-shaped beam.
Electrostatic deflector
The first electrostatic deflector’s main job is beam suppression: to separate the reaction products from the river of unreacted beam particles. To do this, the ions are sent through an electrostatic field that bends particles based on their kinetic energy-to-charge.
The key is that this electrostatic field is set so that the recoils within a target window of kinetic energy-to-charge pass through an exit gap in the deflector, while the unreacted beam ions smash into one of the deflector’s electrodes and are stopped. It’s similar to a car chase in which one car is able to make a sharp turn on a road, but another car with more kinetic energy doesn’t make the turn, and crashes into a wall.
The dipole magnet
As with the electrostatic deflector, the dipole magnet enables EMMA scientists to sift-out ions from one another, in this case using a strong magnetic field to bend particles’ routes based on their momentum-to-charge ratio. This can be done because, in a magnetic field, charged particles will follow a path based on the ratio of their momentum-to-charge. In EMMA, the researchers know the charge state of their experimental recoil products, and the range of their masses, and set the magnet to direct only their range of momentum-to-charge through the exit of the magnet’s vacuum chamber. As a result, all of the ions with a different momentum-to-charge ratio are filtered out.
Second electrostatic deflector
The second electrostatic deflector functions identically to the first, but has a purpose in addition to beam suppression. EMMA scientists also use the second electrostatic deflector to increase the separation between ions of different masses and adjust the angle at which the recoiling ions exit, and thus are focused on the detectors. Furthermore, it completes the energy focusing that was initiated in the dipole magnet, ensuring that after passing through the deflector the trajectories of the ions do not depend on their kinetic energies.
Final quadrupole doublet
As with the first quadrupole doublet, the second focuses the reaction products in the vertical plane. However, unlike with the first doublet, the second is used by EMMA scientists to spread-out the reaction products based on their mass-to-charge ratio. This disperses, or separates, the recoils based on their mass-to-charge ratio in the detector’s focal plane.
For example, an ion with a mass of 84 and charge of +10 will strike the detector in a different area than mass 83 ions with the same charge. As a result, the recoil’s position in the focal plane of the detectors identifies the mass-to-charge ratio, and ions with mass-to-charge ratios differing by 1% can be separated by as much as 20 mm to avoid overlap.
Detectors
Scientists can use up to three EMMA detectors, two of which were custom-made at TRIUMF.
The primary detector is a parallel grid avalanche counter (PGAC), a type of detector specifically developed for experiments with heavy ion beams and focused on measuring a recoil’s location. The PGAC consists of a sandwich of three grids of wires separated by a few mm with a small voltage difference applied between the three layers; these grids are immersed in low-pressure isobutane gas which is contained by very thin (< 1 µm) mylar windows. When a recoil ion passes through it ionizes the gas, producing an avalanche of electrons, an electrical signal that’s collected by the nearest wires. This enables EMMA cientists to measure the positions of the recoils with a precision of one millimeter, which in turn identifies the recoil’s mass-to-charge ratio.
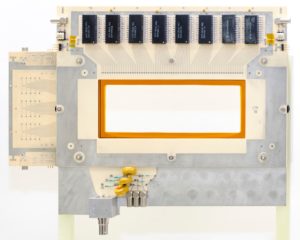
The first detector doesn’t stop the ion, which then enters up to two other detectors. One is a silicon detector that precisely measures the recoil’s energy. The other is an ionization chamber, similar to the parallel grid avalanche counter but operating at up to 20 times the gas pressure. This enables EMMA scientists to infer the number of protons in the nucleus by measuring the ionization produced by the ion as it traverses the chamber.
EMMA is also designed to operate with TIGRESS, a highly sensitive and precise gamma ray detector, to provide essential data on the nuclear reactions being studied. Twelve TIGRESS detectors can be mounted to encircle EMMA’s entire target chamber. The detection of gamma rays with TIGRESS can then be correlated with EMMA’s recoil detections to elucidate the structure of exotic nuclei and the reactions they undergo.